Bactericera cockerelli(PARZCO)
EPPO Datasheet: Bactericera cockerelli
Bactericera cockerelli is a pest in itself (feeding damage), and it transmits ‘Candidatus Liberibacter solanacearum’ to solanaceous plants.
IDENTITY
Authority: (Šulc)
Taxonomic position: Animalia: Arthropoda: Hexapoda: Insecta: Hemiptera: Sternorrhyncha: Triozidae
Other scientific names: Paratrioza cockerelli (Šulc), Trioza cockerelli Šulc
Common names in English: potato psyllid, tomato psyllid
view more common names online...
EPPO Categorization: A1 list
EU Categorization: A1 Quarantine pest (Annex II A)
view more categorizations online...
EPPO Code: PARZCO
HOSTS 2020-10-07
Bactericera cockerelli is found primarily on plants within the family Solanaceae. It attacks, reproduces, and develops on a variety of cultivated and weedy plant species (Essig, 1917; Knowlton & Thomas, 1934; Pletsch, 1947; Jensen, 1954; Wallis, 1955), including crop plants such as potato (Solanum tuberosum), tomato (Solanum lycopersicum), pepper (Capsicum annuum), eggplant (Solanum melongena), and tobacco (Nicotiana tabacum), and non-crop species such as nightshade (Solanum spp.), groundcherry (Physalis spp.) and matrimony vine (Lycium spp.). Adults have been collected from plants in numerous families, including Pinaceae, Salicaceae, Polygonaceae, Chenopodiaceae, Brassicaceae, Asteraceae, Fabaceae, Malvaceae, Amaranthaceae, Lamiaceae, Poaceae, Menthaceae and Convolvulaceae, but this is not an indication of the true host range of this psyllid (Pletsch, 1947; Wallis, 1955; Cranshaw, 1993). In addition to solanaceous species, B. cockerelli has been shown to reproduce and develop on some Convolvulaceae species, including field bindweed (Convolvulus arvensis) and sweet potato (Ipomoea batatas) (Knowlton & Thomas, 1934; List, 1939; Wallis, 1955; Puketapu & Roskruge, 2011; J. E. Munyaneza, unpublished data).
Host list: Capsicum annuum, Chamaesaracha coronopus, Convolvulus arvensis, Datura stramonium, Ipomoea batatas, Lycium barbarum, Lycium berlandieri, Lycium carolineanum, Lycium ferocissimum, Mentha, Micromeria douglasii, Nepeta, Nicandra physalodes, Nicotiana tabacum, Physalis longifolia, Physalis virginiana, Solanum aviculare, Solanum dulcamara, Solanum lycopersicum, Solanum melongena, Solanum pseudocapsicum, Solanum tuberosumGEOGRAPHICAL DISTRIBUTION 2020-10-07
B. cockerelli is thought to be native to South-Western USA and Northern Mexico (Pletsch, 1947; Wallis, 1955). In Canada, this psyllid may survive all year round under protected indoor conditions, but outdoor populations only occur late in the growing season, following the insect migration from Northern Mexico and the USA. B. cockerelli cannot overwinter in Canada and is not considered as established there. In addition, it must be noted that the pathogen ‘Candidatus Liberibacter solanacearum’ has never been observed on potatoes or tomatoes in Canada (Ferguson & Shipp, 2002; Ferguson et al., 2003). In the USA, the potato psyllid had previously been reported to only occur west of the Mississippi River (Richards & Blood, 1933; Pletsch, 1947; Wallis, 1955; Cranshaw, 1993; Capinera, 2001); however, this insect was recently collected on yellow sticky traps near potato fields in Wisconsin late in the summer of 2012 (Henne et al., 2012), which constitutes the first documentation of this insect east of Mississippi. Populations of B. cockerelli were recently found in Ecuador (South America) (Castillo Carrillo et al., 2019).
In New Zealand, B. cockerelli was first detected in May 2006 (Gill, 2006; Teulon et al., 2009; Thomas et al., 2011). The first occurrence in Australia dates back in February 2017 in several private gardens and in a cultivated field in the Perth area (Western Australia). The species was considered under eradication but as early as June 2018, the psyllid was declared not eradicated and a National Plan for the management of the harmful organism was issued (IPPC website. 2017).
Central America and Caribbean: El Salvador, Guatemala, Honduras, Nicaragua
South America: Colombia, Ecuador, Peru
Oceania: Australia (Western Australia), New Zealand, Norfolk Island
BIOLOGY 2020-10-07
The eggs are laid individually on the lower or upper surface of the leaves, usually near the leaf edge, but some eggs can also be found on all above ground parts of suitable host plants. After egg hatching, the young nymphs crawl down the egg stalk to search for a place to feed.
Nymphs are found mostly on the lower surface of the leaves and usually remain sedentary during their entire development preferring sheltered and shaded locations.
Nymphs and adults produce characteristic and large quantities of small whitish excrement which may adhere to foliage and fruit. Adults are more active compared to nymphal stages. They are good fliers and readily jump when disturbed. The pre-oviposition period is normally about 10 days, with oviposition lasting up to 53 days. Total adult longevity ranges from 20 to 62 days and females usually live twice to three times as long as males, depending on which host plants they are reared on (Pletsch, 1947; Abernathy, 1991; Abdullah, 2008; Yang & Liu, 2009).
Females lay an average of 300–500 eggs over their lifetime (Knowlton & Janes, 1931; Pletsch, 1947; Abdullah, 2008; Yang & Liu, 2009). A sex ratio of 1:1 has been reported (Abernathy, 1991; Yang & Liu, 2009). B. cockerelli overwinters as an adult.
Weather is an important element influencing the biology of B. cockerelli and its potential damage to host plants. It seems to be adapted for warm, but not hot weather. Cool weather during migrations, or at least the absence of high temperatures, has been associated with several insect outbreaks (Pletsch, 1947; Wallis, 1955; Capinera, 2001; Cranshaw, 2001).
Optimum psyllid development occurs at approximately 27°C, whereas oviposition, hatching, and survival are reduced at 32°C and stop at 35°C (List, 1939; Pletsch, 1947; Wallis, 1955; Cranshaw, 2001; Abdullah, 2008). A single generation may be completed in 3–5 weeks, depending on temperature. The number of generations varies considerably among regions, usually ranging from three to seven per year. However, once psyllids invade an area, prolonged oviposition by adults may lead to overlapping generations. making it difficult to distinguish them (Pletsch, 1947; Wallis, 1955).
In North America, B. cockerelli appears to migrate annually primarily with wind and hot temperatures in late spring from its overwintering and breeding areas in Western Texas, Southern New Mexico, Arizona, California, and Northern Mexico to northerly regions of the USA and Southern Canada, especially through the mid-western states and Canadian provinces along the Rocky Mountains (Romney, 1939; Pletsch, 1947; Jensen, 1954; Wallis, 1955). In these regions, damaging outbreaks of B. cockerelli in potatoes and tomatoes occurred at regular intervals beginning in the late-1800s and extending into the 1940s (List, 1939; Wallis, 1946; Pletsch, 1947). In more recent years, outbreaks have also occurred in regions outside of the midwestern USA, including in Southern California, Baja California, Washington, Oregon, Idaho, and Central America (Trumble, 2008, 2009; Munyaneza et al., 2009a; Wen et al., 2009; Crosslin et al., 2010, 2012a,b; Munyaneza, 2010, 2012; Butler & Trumble, 2012; Munyaneza & Henne, 2012).
At present, 3 biotypes have been described in USA: western, central and northwestern. Information on B. cockerelli migration movements within Mexico and Central America is lacking. In South-Western USA, potato psyllids reappear in the overwintering areas between October and November, presumably dispersing southward from northern locations (Capinera, 2001); however, their origin has not been determined.
In countries and regions where there is no winter (e.g. Mexico, Central America), temperatures are relatively cool, and suitable host plants may be available for B. cockerelli reproduction and development all year around. It is not known whether migration of this psyllid occurs within New Zealand.
DETECTION AND IDENTIFICATION 2021-01-27
Symptoms
B. cockerelli has historically been associated with psyllid yellows disease of potato and tomato (Richards & Blood, 1933). Psyllid yellows disease is thought to be associated with psyllid nymphs feeding activity (List, 1925, Waters and Darner, 2017, Xia, Qing, 2017) and may be caused by a toxin associated with the insect (Carter, 1939), In the last decade, tomato psyllids have been identified as the vector of ’Candidatus Liberibacter solanacearum’ a bacterial pathogen that causes zebra chip disease in potato and vein greening disease in tomatoes (Prager and Trumble, 2018).
Above the ground, characteristic plant symptoms of infestation by B. cockerelli in potatoes and tomatoes include delayed growth, erectness of new foliage, chlorosis and purpling of new foliage with leaf basal cupping and upward rolling throughout the plant, shortened and thickened terminal internodes resulting in rosetting, enlarged nodes, axillary branches or aerial potato tubers. Additional symptoms are disruption of fruit set, and production of numerous small fruits of poor quality (List, 1939; Pletsch, 1947; Daniels, 1954; Wallis, 1955). Below ground, symptoms on potato include setting of excessive number of tiny misshaped potato tubers, production of chain tubers, and early breaking of tuber dormancy (List, 1939; Pletsch, 1947; Wallis, 1955).
Additional potato tuber symptoms associated with transmission of ‘Candidatus Liberibacter solanacearum’ include collapsed stolons, browning of vascular tissue in combination with necrotic flecking of internal tissues and streaking of the medullary ray tissues, all of which can affect the entire tuber. Upon frying, these symptoms become more pronounced and crisps or chips processed from affected tubers show very dark blotches, stripes, or streaks, rendering them commercially unacceptable (Munyaneza et al., 2007a,b, 2008; Secor et al., 2009; Crosslin et al., 2010; Miles et al., 2010; Munyaneza, 2012; Munyaneza & Henne, 2012).
Morphology
Eggs
Eggs are oval and are connected to the leaf surface by a thin stalk. The eggs initially are light-yellow, but with time turn dark-yellow or orange. The egg measures about 0.32–0.34 mm long, 0.13–0.15 mm wide, and with a stalk of 0.48–0.51 mm. Eggs hatch 3–7 days after oviposition (Pletsch, 1947; Wallis, 1955; Capinera, 2001; Abdullah, 2008; Butler & Trumble, 2012; Munyaneza, 2012; Munyaneza & Henne, 2012).
Nymphs
Nymphs are elliptical when viewed from above, but very flattened in profile, appearing almost scale-like. B. cockerelli nymphs can also be confused with nymphs of whiteflies, although the former move when disturbed. Five nymphal instars can be found, each instar having very similar morphological features other than size. Nymphal body width is variable, ranging from 0.23 to 1.60 mm, depending on different instars (Rowe & Knowlton, 1935; Pletsch, 1947; Wallis, 1955; Butler & Trumble, 2012; Munyaneza, 2012; Munyaneza & Henne, 2012). Nymphs are initially orange, become yellowish-green and then green as they mature. The compound eyes are reddish and quite prominent.
During the third instar, the wing pads which are light in colour become evident and get more pronounced with each molt. A short fringe of wax filaments is present along the lateral margins of the body. Total nymphal development time ranges of 12–24 days depending on temperature and host plant (Knowlton & Janes, 1931; Abdullah, 2008; Yang & Liu, 2009).
Adult
The adults are quite small, measuring about 2.5–2.75 mm long, resembling tiny cicadas, largely because their wings are held angled and roof-like over their body (Wallis, 1955; Butler & Trumble, 2012; Munyaneza, 2012; Munyaneza & Henne, 2012). They have two pairs of clear wings, front wings being considerably larger than the hind ones. The antennae are moderately long, about the length of the thorax. Body colour ranges from pale green at emergence, to dark green or brown within 2–3 days, and grey or black thereafter. White or yellow lines are found on the head and thorax, and whitish bands on the first and terminal abdominal segments. These white markings, particularly the broad, transverse white band on the first abdominal segment and the inverted V-shaped white mark on the last abdominal segment, are distinguishing characteristics of B. cockerelli (Pletsch, 1947; Wallis, 1955).
Molecular
Accurate and rapid identification of pests upon first occurrence in a new area is essential to prevent spread to areas where they do not occur and to control outbreaks. B. cockerelli is considered a serious threat for the EPPO region so it is necessary to develop and validate diagnostic tests that allow rapid identification. Sumner-Kalkun et al., (2020) designed and validated the first specific real-time PCR based on a TaqMan probe, targeting the region of the ITS2 gene of B. cockerelli. The test can be performed on DNA extracted from a single adult, juvenile stage- and egg. Its exclusivity was evaluated on 73 species of psyllids collected in various areas of Europe, New Zealand, Mexico and the United States including in the genus Bactericera other than B. cockerelli and the main vectors of ‘Candidatus Liberibacter solanacearum’ worldwide (Summer-Kalkun et al., 2020).
‘Candidatus Liberibacter solanacearum’ is present in numerous organs and tissues of B. cockerelli, including the alimentary canal, salivary glands, hemolymph and bacteriomas. The presence of the bacterium 'Candidatus Liberibacter solanacearum’ in vector insects can be reliably detected by conventional and real time PCR in samples of 10 individuals of B. cockerelli collected in the field by the use of yellow chromotropic traps or by manual collection. Details on testing on vectors are provided in PM 7/143 (EPPO, 2020a) (Cooper et al., 2013; Crosslin et al., 2011).
PATHWAYS FOR MOVEMENT 2020-10-07
Adults of B. cockerelli are good fliers and can disperse over considerable distances with the onset of wind and hot temperatures. Adults have been shown to migrate massively to northern and western states of the USA and southern Canadian provinces in the spring from the insect overwintering sites in the South-Western USA and Northern Mexico (i.e. several hundred km). Immature stages of B. cockerelli are essentially sedentary and do not actively disperse. Long distance transport of different life stages of this pest is possible, mainly by commercial trade of plants of Solanaceae family, which represent major hosts for B. cockerelli. This pest was introduced into New Zealand probably by the transport of plant material from Western USA, possibly as eggs (Crosslin et al., 2010; Thomas et al., 2011). Entry on fruit of host species (e.g. tomato, pepper) is also possible, especially when they are associated with green parts (e.g. truss tomato). No life stages of B. cockerelli are associated with potato tubers or soil.
PEST SIGNIFICANCE 2021-01-27
Economic impact
The extensive damage to solanaceous crops that was historically observed during the outbreak years of the early 1900s in Mid-Western USA is thought to have been due to B. cockerelli’s association with a physiological disorder in plants referred to ‘psyllid yellow’. Infected tomato plants produced few or no marketable fruit (List, 1939; Daniels, 1954). In potatoes, psyllid yellows lead to yellowing or purpling of foliage, early death of plants, and low yields of marketable tubers (Eyer, 1937; Pletsch, 1947; Daniels, 1954; Wallis, 1955). In areas of outbreaks of psyllid yellows, the disorder was often present in 100% of plants in affected fields, with yield losses exceeding 50% in some areas (Pletsch, 1947). Many of the outbreaks in the early 1900s occurred well north of the insect’s overwintering range, such as the states of Montana and Wyoming (Pletsch, 1947), proving the dispersal capabilities of the psyllid.
Recently, potato, tomato, and pepper growers in a number of geographic areas have suffered extensive economic losses associated with outbreaks of B. cockerelli (Trumble, 2008, 2009; Munyaneza et al., 2009b,c,d; Crosslin et al., 2010; Munyaneza, 2010, 2012; Butler & Trumble, 2012; Munyaneza & Henne, 2012). This increased damage is due to a previously undescribed species of the bacterium Liberibacter, tentatively named ‘Candidatus Liberibacter solanacearum’ (syn. ‘Ca. L. psyllaurous’) (Hansen et al., 2008; Liefting et al., 2009), now known to be vectored by B. cockerelli (Munyaneza et al., 2007a,b; Buchman et al., 2011a,b; Munyaneza, 2012, Sengoda et. al., 2014). Potato psyllids acquire and spread the pathogen by feeding on infected plants (Munyaneza et al., 2007a,b; Buchman et al., 2011a,b). The bacterium is also transmitted transovarially in the psyllid (Hansen et al., 2008), which contributes to spread the disease between geographic regions by dispersing psyllids and helps maintain the bacterium in geographic regions during the insect’s overwintering period (Crosslin et al., 2010; Munyaneza, 2012). Symptoms associated with Liberibacter in tomatoes and pepper include chlorosis and purpling of leaves, leaf scorching, stunting or death of plants, and production of small, poor-quality fruit (Liefting et al., 2009; McKenzie & Shatters, 2009; Munyaneza et al., 2009c,d; Brown et al., 2010; Crosslin et al., 2010; Butler & Trumble, 2012). During the outbreaks of 2001–2003, tomato growers in coastal California and Baja California suffered losses exceeding 50–80% of the crop (Trumble, 2009; Butler & Trumble, 2012). In potatoes, Liberibacter foliar symptoms closely resemble those caused by psyllid yellows and purple top diseases (Munyaneza et al., 2007a,b; Sengoda et al., 2010).
However, tubers from Liberibacter-infected plants develop a defect referred to as ‘zebra chip’, which is not induced by the potential toxin causing psyllid yellows (Munyaneza et al., 2007a,b, 2008; Sengoda et al., 2009; Wenninger et al, 2017). Tubers show a striped pattern of necrosis, which is particularly noticeable when the tuber is processed for crisps or chips (Munyaneza et al., 2007a,b, 2008; Miles et al., 2010; Wenninger et.al, 2017). When affected by zebra chip, potato tubers become unsuitable for processing and cannot be used in the fresh market as both the taste and the internal appearance of the tuber are altered (Vereijssen et al., 2018). Such defect was of sporadic importance until 2004, when it began to cause millions of dollars in losses to potato growers in the USA, Central America, and Mexico (Rubio-Covarrubias et al., 2006; Munyaneza et al., 2007a, 2009b; Crosslin et al., 2010; Munyaneza, 2010, 2012; Munyaneza & Henne, 2012). In some regions, entire fields have been abandoned because of zebra chip (Secor & Rivera-Varas, 2004; Munyaneza et al., 2007a; Crosslin et al., 2010; Munyaneza, 2010, 2012; Munyaneza & Henne, 2012). The potato industry in Texas estimates that zebra chip could affect over 35% of the potato acreage in Texas, with potential losses annually to growers exceeding 25 million USD (CNAS, 2006). Finally, quarantine issues have begun to emerge in potato psyllid-affected regions because some countries now require that shipments of solanaceous crops from certain growing regions are tested for the pathogen before the shipments are allowed entry (Crosslin et al., 2010; Munyaneza, 2012; Wenninger et al., 2017).
The impact of B. cockerelli and ‘Candidatus Liberibacter solanacearum’ on agriculture is very high. Since their arrival in New Zealand in 2005, it has caused millions of dollars in economic losses in terms of production losses, increased crop management costs and market losses export (Teulon et al., 2009). The introduction of B. cockerelli into potato growing regions in Europe or Asia would have devastating effects on the agricultural industry in those regions. If B. cockerelli were to invade Europe, it is estimated that they could cause damage to the potato and tomato sector of 222 million EUR per year (Soliman, 2012).
A global scale study comparing land-use maps and environments suitable for B. cockerelli / ‘Candidatus Liberibacter solanacearum’, showed that of the nearly 80% of the global potato production area (96 % of the potato growing area in South America, 14 % in Eurasia, 100 % in Australia) can be considered at risk of damage (Wan et al., 2020).
Control
Monitoring B. cockerelli is essential for effective management of this insect pest. Early season management is crucial to minimize damage and psyllid reproduction in the field. The adult populations are commonly sampled using sweep nets or vacuum devices, but egg and nymphal sampling requires visual examination of foliage. The adults can also be sampled with yellow water-pan traps. Typically, psyllid populations are highest at field edges initially, but if not controlled, the insects will eventually spread throughout the crop (Henne et al., 2010; Butler & Trumble, 2012; Workneh et al., 2012).
Bactericera cockerelli control is currently achieved by insecticide applications (Goolsby et al., 2007; Gharalari et al., 2009; Berry et al., 2009; Butler et al., 2011; Munyaneza, 2012; Butler & Trumble, 2012; Munyaneza & Henne, 2012; Guenthner et al., 2012) but psyllids have been shown to easily develop insecticide resistance due to their high fecundity and short generation times (McMullen & Jong, 1971).
Therefore, alternative strategies should be considered to limit the impact of B. cockerelli and its associated diseases. Even with conventional insecticides, B. cockerelli tends to be difficult to manage. It has been determined that Liberibacter is transmitted to potato very rapidly by the potato psyllid and a single psyllid per plant can successfully transmit this bacterium to potato in 6 hours, leading to zebra chip disease (Buchman et al., 2011a,b).Just a few infectious psyllids feeding on potato for a short period can result in substantial spread of the disease within a potato field or region (Henne et al., 2010).
Conventional pesticides may have limited direct disease control as they may not kill the potato psyllid quickly enough to prevent bacterial transmission, although they may be useful for reducing the overall population of psyllids. The most valuable and effective strategies to manage zebra chip disease would likely be those that discourage vector feeding, such as use of plants that are resistant to psyllid feeding or less preferred by the psyllid. Unfortunately, no potato variety has so far been shown to exhibit sufficient resistance or tolerance to zebra chip or potato psyllid (Munyaneza et al., 2011).
However, some conventional and biorational pesticides, including plant and mineral oils and kaolin, have shown some substantial deterrence and repellency to potato psyllid feeding and oviposition (Gharalari et al., 2009; Yang et al., 2010; Butler et al., 2011; Peng et al., 2011) and could be useful tools in integrated pest management programs to manage zebra chip and its psyllid vector
Good insecticide coverage or translaminar activity is important because psyllids are commonly found on the underside of the leaves. Insecticides controlling adults do not necessary controls nymphs or eggs. Active ingredients used in the USA against B. cockerelli include imidacloprid, thiamethoxam, spiromesifen, dinotefuran, pyriproxyfen, pymetrozine, and abamectin (Goolsby et al., 2007; Liu & Trumble, 2007; Liu et al., 2006; Butler & Trumble, 2012; Munyaneza & Henne, 2012; Guenthner et al., 2012). In New Zealand, the list of products to control B. cockerelli includes acephate, metamidophos, imidacloprid, thiacloprid, buprofezin, abamectin, cypermethrin, deltamethrin, lambda-cyhalothrin, esfenvalerate, spinosad, and spirotetramat (Berry et al., 2009).
The frequent use of neonicotinoids (group 4A) throughout the southwestern United States to control B. cockerelli has raised concerns about widespread resistance to such actives. By exposing the young nymphs collected in the field at different dose of two neonicotinoids, imidacloprid and thiamethoxam, it was verified that they developed resistant to both insecticides and the level of resistance depending on the geographical position of psyllids tested. Such results underline the need to diversify and integrate the strategies used in B. cockerelli integrated management of and reduce dependence on insecticides to manage psyllid infestations and disease (Szczepaniec et al., 2019).
In the pest control strategies, it may be necessary to employ different insecticides, although this is in contrast with anti-resistance strategies (EPPO, 2020b).
B. cockerelli is affected by a large number of natural enemies, including chrysopid larvae, ladybirds, geocorids, anthocorids, myrids, nabids, syrphid larvae and the parasitoids Tamarixia triozae (Hymenoptera: Eulophidae) and Metaphycus psyllidis (Hymenoptera), known for their effects on psyllid populations (Pletsch, 1947; Wallis, 1955; Cranshaw, 1993, Butler et al., 2010; Butler and Trumble, 2012a; Liu et al., 2012). In addition, several entomopathogenic fungi, including Beauveria bassiana, Metarhizium anisopliae and Isaria fumosorosea, proved to be effective natural enemies of B. cockerelli, causing laboratory and field mortality of up to 99 and 78% respectively (Lacey et al., 2009; 2011).
In New Zealand 16 native strains of entomopathogenic fungi used against adults and nymphs of B. cockerelli were selected. In laboratory tests, a strain of Beauveria bassiana (ICMP 8701) resulted in high mortality in first instar nymphs and young adults. These results allow to consider B. bassiana ICMP 8701 as a component in the integrated management of the B. cockerelli in New Zealand- (Liu et al., 2020). Tamarixia triozae (Burks) is an important parasitoid of B. cockerelli which has been evaluated for use as a biological control agent of the psyllid (Rojas et al., 2014). In New Zealand the release of the psyllid parasitoid Tamarixia triozae has been recently approved to assist with the biological control of B. cockerelli in field crops (EPA, 2016), as well Amblydromalus limonicus, a predatory mite for use in greenhouses (EPPO, 2020b).
Phytosanitary risk
Bactericera cockerelli has been found to be a serious and economically important pest in potatoes, tomatoes, and other solanaceous crops in the Western USA, Mexico, Central America and New Zealand, because of its direct feeding impact and as a vector of ‘Candidatus Liberibacter solanacearum’ (Guenthner et al., 2012; Munyaneza, 2012). Given the impact of B. cockerelli in regions where it occurs, its introduction in the EPPO region would have massive negative impacts, especially if the insects were carrying ‘Candidatus Liberibacter solanacearum’. Suitable host plants are widespread in the region and, given its current distribution in the Americas and New Zealand, it is thought that B. cockerelli would be able to establish and overwinter outdoors in the Southern and Central European part of EPPO region, as well as in areas with mild winters in the Northern part of the region, comparable to those of the South Island in New Zealand. It could also establish under protected conditions in the entire EPPO region. Moreover, the migratory behaviour of B. cockerelli which favours rapid and long-distance dispersal would put the EPPO region at a high risk, if the psyllid was introduced.
PHYTOSANITARY MEASURES 2021-01-27
Suggested phytosanitary measures are specified in the PRA performed by EPPO (EPPO, 2012); they are as follows. All commodities should be accompanied by a Phytosanitary Certificate (PC) and if appropriate a PC of re-export. Fruits of Solanaceae should originate from an area free from B. cockerelli or from a pest-free site under complete physical isolation. For tomato only, a Systems Approach is also possible and should include that the fruits are grown under protected conditions, green parts are removed, fruits are washed and fumigated, and consignment inspected. Import of plants for planting of Solanaceae (including potatoes) is prohibited in many EPPO countries. However, if import is considered, the following measures should be considered. Plants for planting of Solanaceae (except seeds) and living parts of Solanaceae (e.g. cut flowers) should originate from an area free from the pest and from ‘Ca. L. solanacearum’. Seed potato (including microplants and minitubers) should originate from an area free from ‘Ca. L. solanacearum’ in Solanaceae and ware potatoes should originate from an area free from the pest and ‘Ca. L. solanacearum’ in Solanaceae in production. Alternatively, high grade seed potato may be imported under post-entry quarantine, and ware potatoes may be imported for industrial processing purposes. Plants for planting of non-Solanaceous hosts of B. cockerelli (e.g. Micromeria chamissonis, Mentha spp., Nepeta spp. and Ipomoea batatas) should originate from an area free from the pest and from ‘Ca. L. solanacearum’.
In the event of an outbreak of B. cockerelli (with or without haplotypes A or B of ‘Ca. L. solanacearum’) in an EPPO country, appropriate eradication measures by delimiting the infested area and defining buffer areas around outbreaks are recommended (EPPO, 2020b).
In 2011, before the arrival of B. cockerelli on their territory, the Australian authorities (Plant Health Australia) had already developed a management plan based on the application of some insecticides capable of protecting potato crops in the different stages of their development (EPPO, 2020). This plan was subsequently adopted in 2018 when B. cockerelli was found and deemed no longer eradicable (IPPC website, 2020). EPPO countries can prepare a contingency plan for B. cockerelli (EPPO, 2020b).
REFERENCES 2021-01-27
Abdullah NMM (2008) Life history of the potato psyllid Bactericera cockerelli (Homoptera: Psyllidae) in controlled environment agriculture in Arizona. African Journal of Agricultural Research 3, 60–67.
Abernathy RL (1991) Investigation into the nature of the potato psyllid toxin. MS Thesis. Colorado State University, Fort Collins, CO. Berry NA, Walker MK & Butler RC (2009) Laboratory studies to determine the efficacy of selected insecticides on tomato/potato psyllid. New Zealand Plant Protection 62, 145–151.
Brown JK, Rehman M, Rogan D, Martin RR & Idris AM (2010) First report of 'Candidatus Liberibacter psylaurous' (syn. 'Ca. L. solanacearum') associated with the ‘tomato vein-greening’ and ‘tomato psyllid yellows’ diseases in commercial greenhouse in Arizona. Plant Disease 94, 376.
Buchman JL, Heilman BE & Munyaneza JE (2011a) Effects of Bactericera cockerelli (Hemiptera: Triozidae) density on zebra chip potato disease incidence, potato yield, and tuber processing quality. Journal of Economic Entomology 104, 1783–1792.
Buchman JL, Sengoda VG & Munyaneza JE (2011b) Vector transmission efficiency of liberibacter by Bactericera cockerelli (Hemiptera: Triozidae) in zebra chip potato disease: effects of psyllid life stage and inoculation access period. Journal of Economic Entomology 104, 1486–1495.
Butler CD, Byrne FR, Keremane ML, Lee RF & Trumble JT (2010) Effects of insecticides on behavior of adult Bactericera cockerelli (Hemiptera: Triozidae) and transmission of Candidatus Liberibacter psyllaurous. Journal of Economic Entomology 104, 586–594.
Butler CD & Trumble JT (2012) The potato psyllid, Bactericera cockerelli (Sulc) (Hemiptera: Triozidae): life history, relationship to plant diseases, and management strategies. Terrestrial Arthropod Reviews 5, 87–111.
Capinera JL (2001) Handbook of Vegetable Pests. Academic Press, San Diego, CA. Carter W (1939) Injuries to plants caused by insect toxins. Botanical Review 5, 273–326.
Castillo Carrillo C, Fu Z, Burckhardt D (2019) First record of the tomato potato psyllid Bactericera cockerelli from South America. Bulletin of Insectology 72 (1), 85-91.
CNAS (2006) Economic Impacts of Zebra Chip on the Texas Potato Industry. Center for North American Studies. Available at: http://cnas.tamu.edu/zebra%20chip%20impacts%20final.pdf [last accessed October 2020]
Cranshaw WS (1993) An annotated bibliography of potato/tomato psyllid, Paratrioza cockerelli (Sulc) (Homptera; Psyllidae). Colorado State University Agricultural Experiment Station Bulletin, TB93–5.
Cranshaw WS (2001) Diseases caused by insect toxin: psyllid yellows. In: Compendium of Potato Diseases, 2nd edn (Eds. Stevenson WR, Loria R, Franc GD & Weingartner DP), pp. 73–74, APS Press St. Paul, MN.
Crosslin JM, Munyaneza JE, Brown JK & Liefting LW (2010) Potato zebra chip disease: a phytopathological tale. Online. Plant Health Progress. https://doi.org/10.1094/PHP-2010-0317-01-RV
Cooper WR, Sengoda VG & Munyaneza JE (2013) Localization of ‘Candidatus Liberibacter solanacearum’ in Bactericera cockerelli (Hemiptera: Triozidae). Annals of the Entomological Society of America 107, 204– 210.
Crosslin JM, Lin H & Munyaneza JE (2011) Detection of ‘Candidatus Liberibacter solanacearum’ in the potato psyllid, Bactericera cockerelli (Šulc), by conventional and real‐time PCR. Southwestern Entomologist 36, 125– 135.
Daniels LB (1954) The nature of the toxicogenic condition resulting from the feeding of the tomato psyllid Paratrioza cockerelli (Sulc), PhD Dissertation, University of Minnesota.
EPPO (2020a) PM 7/143 Diagnostic Standard ‘Candidatus Liberibacter solanacearum’. EPPO Bulletin 50(1), 49-68.
EPPO (2020b) PM 9/25 National Regulatory Control Standard Bactericera cockerelli and ‘Candidatus Liberibacter solanacearum’. EPPO Bulletin 50(3), 496-509.
EPPO (2012) Final pest risk analysis for Bactericera cockerelli. EPPO, Paris.
Essig EO (1917) The tomato and laurel psyllids. Journal of Economic Entomology 10, 433–444.
Eyer JR (1937) Physiology of psyllid yellows of potatoes. Journal of Economic Entomology 30, 891–898.
JF Liu, ZQ Zhang, JR Beggs, E Paderes, X Zou, XY Wei (2020) Lethal and sublethal effects of entomopathogenic fungi on tomato/potato psyllid, Bactericera cockerelli (Šulc) (Hemiptera: Triozidae) in capsicum. Crop Protection 129, 105023
Ferguson G & Shipp L (2002) New pests in Ontario greenhouse vegetables. Working Group 'International Control in Protected Crops, Temperate Climate'. Bulletin OILB/SROP 25, 69–72.
Ferguson G, Banks E & Fraser H (2003) B. cockerelli in Canada potato psyllid – a new pest in greenhouse tomatoes and peppers. OMAF. http://www.omafra.gov.on.ca/english/crops/facts/potato_psyllid.htm [accessed on 26 October 2010].
Gharalari AH, Nansen C, Lawson DS, Gilley J, Munyaneza JE & Vaughn K (2009) Knockdown mortality, repellency, and residual effects of insecticides for control of adult Bactericera cockerelli (Hemiptera: Psyllidae). Journal of Economic Entomology 102, 1032–1038.
Gill G (2006) Tomato psyllid detected in New Zealand. Biosecurity New Zealand 69, 10–11.
Goolsby JA, Adamczyk J, Bextine B, Lin D, Munyaneza JE & Bester G (2007) Development of an IPM program for management of the potato psyllid to reduce incidence of zebra chip disorder in potatoes. Subtropical Plant Science 59, 85–94.
Guenthner J, Goolsby J & Greenway G (2012) Use and cost of insecticides to control potato psyllids and zebra chip on potatoes. Southwestern Entomologist 37, 263–270.
Hansen AK, Trumble JT, Stouthamer R & Paine TD (2008) A new huanglongbing species, ‘Candidatus Liberibacter psyllaurous’ found to infect tomato and potato, is vectored by the psyllid Bactericera cockerelli (Sulc). Applied Environmental Microbiology 74, 5862– 5865.
Henne DC, Workneh F & Rush CM (2010) Movement of Bactericera cockerelli (Heteroptera: Psyllidae) in relation to potato canopy structure, and effects of potato tuber weights. Journal of Economic Entomology 103, 1524–1530.
Henne DC, Anciso J, Bradshaw J, Whipple S, Carpio L, Schuster G et al. (2012) Overview of the 2011–2012 potato psyllid area wide monitoring program. In: Proceedings of the 2012 Annual Zebra Chip Reporting Session (Ed. Workneh F, Rashed A & Rush CM), pp. 1–5 San Antonio, TX (October 30–November 2, 2012).
IPPC website. Official Pest Reports – Australia (AUS-78/1 of 2017-02-16)
Jackson BC, Goolsby J, Wyzykowski A, Vitovksy N & Bextine B (2009) Analysis of genetic relationship between potato psyllid (Bactericera cockerelli) populations in the United States, Mexico, and Guatemala using ITS2 and inter simple sequence repeat (ISSR) data. Subtropical Plant Science 61, 1–5.
Jensen DD (1954) Notes on the potato psyllid, Paratrioza cockerelli (Sulc) (Hemiptera: Psyllidae). Pan-Pacific Entomologist 30, 161–165.
Knowlton GF & Janes MJ (1931) Studies on the biology of Paratrioza cockerelli (Sulc). Annals of the Entomological Society of America 24, 283–291.
Knowlton GF & Thomas WL (1934) Host plants of the potato psyllid. Journal of Economic Entomology 27, 547.
Lacey LA; Liu TX; Buchman JL; Munyaneza JE; Goolsby JA; Horton DR, (2011). Entomopathogenic fungi (Hypocreales) for control of potato psyllid, Bactericera cockerelli (Sulc) (Hemiptera: Triozidae) in an area endemic for zebra chip disease of potato. Biological Control, 56(3), 271-278
Liefting LW, Sutherland PW, Ward LI, Paice KL, Weir BS & Clover GRG (2009) A new ‘Candidatus Liberibacter’ species associated with diseases of solanaceous crops. Plant Disease 93, 208–214.
List GM (1925) The tomato psyllid, Paratrioza cockerelli Sulc. Colorado State Entomologist Circular, 47.
List GM (1939) The effect of temperature upon egg deposition, egg hatch and nymphal development of Paratrioza cockerelli (Sulc). Journal of Economic Entomology 32, 30–36.
Liu D & Trumble JT (2007) Comparative fitness of invasive and native populations of the potato psyllid (Bactericera cockerelli). Entomologia Experimentalis et Applicata 123, 35–42.
Liu D, Trumble JT & Stouthamer R (2006) Genetic differentiation between eastern populations and recent introductions of potato psyllid (Bactericera cockerelli) into western North America. Entomologia Experimentalis et Applicata 118, 177–183.
McKenzie CL & Shatters RG Jr (2009) First report of 'Candidatus Liberibacter psyllaurous' associated with psyllid yellows of tomato in Colorado. Plant Disease 93, 1074.
McMullen RD & Jong C (1971) Dithiocarbamate fungicides for control of pear psylla. Journal of Economic Entomology 64, 1266–1270.
Miles GP, Samuel MA, Chen J, Civerolo EL & Munyaneza JE (2010) Evidence that cell death is associated with zebra chip disease in potato tubers. American Journal of Potato Research 87, 337–349.
Munyaneza JE (2010) Psyllids as vectors of emerging bacterial diseases of annual crops. Southwestern Entomologist 35, 417–477.
Munyaneza JE (2012) Zebra chip disease of potato: biology, epidemiology, and management. American Journal of Potato Research 89, 329–350.
Munyaneza JE & Henne DC (2012) Leafhopper and psyllid pests of potato. In: Insect Pests of Potato: Global Perspectives on Biology and Management (Ed. Giordanengo P, Vincent C & Alyokhin A), pp. 65–102. Academic Press, San Diego, CA.
Munyaneza JE, Crosslin JM & Upton JE (2007a) Association of Bactericera cockerelli (Homoptera: Psyllidae) with 'zebra chip', a new potato disease in southwestern United States and Mexico. Journal of Economic Entomology 100, 656–663.
Munyaneza JE, Goolsby JA, Crosslin JM & Upton JE (2007b) Further evidence that zebra chip potato disease in the lower Rio Grande Valley of Texas is associated with Bactericera cockerelli. Subtropical Plant Science 59, 30–37.
Munyaneza JE, Buchman JL, Upton JE, Goolsby JA, Crosslin JM, Bester G et al. (2008) Impact of different potato psyllid populations on zebra chip disease incidence, severity, and potato yield. Subtropical Plant Science 60, 27–37.
Munyaneza JE, Buchman JL & Crosslin JM (2009a) Seasonal occurrence and abundance of the potato psyllid, Bactericera cockerelli, in South Central Washington. American Journal for Potato Research 86, 513–518.
Munyaneza JE, Sengoda VG, Crosslin JM, De la Rosa-Lozano G & Sanchez A (2009b) First report of ‘Candidatus Liberibacter psyllaurous’ in potato tubers with zebra chip disease in Mexico. Plant Disease 93, 552.
Munyaneza JE, Sengoda VG, Crosslin JM, Garzon-Tiznado J & Cardenas-Valenzuela O (2009c) First report of ‘Candidatus Liberibacter solanacearum’ in tomato plants in Mexico. Plant Disease 93, 1076.
Munyaneza JE, Sengoda VG, Crosslin JM, Garzon-Tiznado J & Cardenas-Valenzuela O (2009d) First report of ‘Candidatus Liberibacter solanacearum’ in pepper in Mexico. Plant Disease 93, 1076.
Munyaneza JE, Buchman JL, Sengoda VG, Goolsby JA, Ochoa AP, Trevino J et al (2012) Impact of potato planting time on incidence of potato zebra chip disease in the Lower Rio Grande Valley of Texas. Southwestern Entomologist 37, 253–262.
Munyaneza JE, Buchman JL, Sengoda VG, Fisher TW & Pearson CC (2011) Susceptibility of selected potato varieties to zebra chip potato disease. American Journal of Potato Research 88, 435–440.
Peng L, Trumble JT, Munyaneza JE & Liu TX (2011) Repellency of a kaolin particle film to potato psyllid, Bactericera cockerelli (Hemiptera: Psyllidae), on tomato under laboratory and field conditions. Pest Management Science 67, 815–824.
Pletsch DJ (1947) The potato psyllid Paratrioza cockerelli (Sulc) its biology and control. Montana Agricultural Experiment Station Bulletin 446, 95.
Puketapu A & Roskruge, N (2011) The tomato-potato psyllid lifecycle on three traditional Maori food sources. Agronomy New Zealand 41, 167–173.
Prager SM, Trumble JT (2018) - Sustainable management of arthropod pests of tomato – Chapter 7 Psyllids: Biology, Ecology, and Management. Elsevier https://doi.org/10.1016/B978-0-12-802441-6.00007-3
Richards BL & Blood HL (1933) Psyllid yellows of the potato. Journal of Agricultural Research 46, 189–216.
Rojas P., Rodríguez-Leyva E., J. Lomeli-Flores R. & Xian Liu T. (2014) Biology and life history of Tamarixia triozae, a parasitoid of the potato psyllid Bactericera cockerelli. BioControl 60, 27–35.
Romney VE (1939) Breeding areas of the tomato psyllid, Paratrioza cockerelli (Sulc). Journal of Economic Entomology 32, 150–151.
Rowe JA & Knowlton GF (1935) Studies upon the morphology of Paratrioza cockerelli (Sulc). Journal of Utah Academic Science 12, 233–237.
Rubio-Covarrubias OA, Almeyda-Leon IH, Moreno JI, Sanchez-Salas JA, Sosa RF, Borbon-Soto JT, Hernandez CD, Garzon-Tiznado JA,
Rodriguez RR & Cadena-Hinajosa MA (2006) Distribution of potato purple top and Bactericera cockerelli Sulc. in the main potato production zones in Mexico. Agricultura Tecnica en Mexico 32, 201–211.
Secor GA & Rivera-Varas V (2004) Emerging diseases of cultivated potato and their impact on Latin America. Revista Latinoamericana de la Papa (Suplemento) 1, 1–8.
Secor GA, Rivera VV, Abad JA, Lee IM, Clover GRG, Liefting LW et al (2009) Association of ‘Candidatus Liberibacter solanacearum’ with zebra chip disease of potato established by graft and psyllid transmission, electron microscopy, and PCR. Plant Disease 93, 574–583.
Sengoda VG, Cooper WR, Swisher KD, Henne DC & Munyaneza JE (2014) Latent period and transmission of ‘Candidatus Liberibacter solanacearum’ by the potato psyllid Bactericera cockerelli (Hemiptera: Triozidae). PLoS ONE 9(3), e93475.
Soliman T (2012) Economic impact assessment of invasive plant pests in the European Union. Wageningen University, Wageningen, Netherlands.
Summer Kalkun JC, Sjölund MJ, Arnsdorf YM, Carnegie M, Highet F, Ouvrard D, Greenslade AFC, Bell JR, Sigvald R., Kenyon DM (2020) A diagnostic real-time PCR assay for the rapid identification of the tomato-potato psyllid, Bactericera cockerelli (Šulc, 1909) and development of a psyllid barcoding database. Plos ONE 5(3), e0230741. https://doi.org/10.1371/journal.pone.0230741
Szczepaniec A, Varela KA, Kiani M, Paetzold L (2019) Incidence of resistance to neonicotinoid insecticides in Bactericera cockerelli across Southwest U.S. Crop Protection, 116, 188-195.
Teulon DAJ, Workman PJ, Thomas KL & Nielsen MC (2009) Bactericera cockerelli: incursion, dispersal and current distribution on vegetable crops in New Zealand. New Zealand Plant Protection 62, 136–144.
Thomas KL, Jones DC, Kumarasinghe LB, Richmond JE, Gill GSC & Bullians MS (2011) Investigation into the entry pathway for the tomato potato psyllid Bactericera cockerelli. New Zealand Plant Protection 64, 259–268.
Trumble J (2009) Potato Psyllid. Center for Invasive Species Research, University of California, Riverside. Available at: https://cisr.ucr.edu/invasive-species/potato-psyllid [last accessed October 2020].
Vereijssen J, Smith GR, Weintraub PG (2018) Bactericera cockerelli (Hemiptera: Triozidae) and 'Candidatus Liberibacter solanacearum’ in Potatoes in New Zealand: Biology, Transmission, and Implications for Management. Journal of Integrated Pest Management 9, 1-21.
Wallis RL (1946) Seasonal occurrence of the potato psyllid in the North Platte Valley. Journal of Economic Entomology 39, 689–694.
Wallis RL (1955) Ecological studies on the potato psyllid as a pest of potatoes. USDA Technical Bulletin, 1107.
Wan J, Wang R, Ren Y, McKirdy S (2020) Potential distribution and the risks of Bactericera cockerelli and its associated plant pathogen Candidatus Liberibacter solanacearum’ for global potato production. Insects 11(5), 298. https://doi.org/10.3390/insects11050298
Waters T.D., Darner J.K. (2017) Management of Potato Psyllid Nymphs on Potato, 2016. Arthropod Management Tests 42, 1.
Wen A, Mallik I, Alvarado VY, Pasche JS, Wang X, Li W et al. (2009) Detection, distribution, and genetic variability of ‘Candidatus Liberibacter’ species associated with zebra complex disease of potato in North America. Plant Disease 93, 1102–1115.
Wenninger EJ, Carroll A, Dahan J, Karasev AV, Thornton M, Miller J, Nolte P, Olsen P, Price W (2017) Phenology of the potato psyllid, Bactericera cockerelli (Hemiptera: Triozidae), and 'Candidatus Liberibacter solanacearum’ in commercial potato fields in Idaho. Environmental Entomology 46, 1179–1188.
Workneh F, Henne DC, Childers AC, Paetzold L & Rush CM (2012) Assessments of the edge effects in intensity of potato zebra chip disease. Plant Disease 96, 943–947.
Xia, Qing (2017) Analysis of historical and current distribution of potato psyllid (Bactericera cockerelli) and the induced plant disease psyllid yellows, in relation to standard climate indices. PhD Thesis, University of Lethbridge Research Repository, https://hdl.handle.net/10133/4924
Yang XB & Liu TX (2009) Life history and life tables of Bactericera cockerelli (Homoptera: Psyllidae) on eggplant and bell pepper. Journal of Environmental Entomology 38, 1661–1667.
Yang XB, Zhang YM, Hau L, Peng LN, Munyaneza JE & Liu TX (2010) Repellency of selected biorational insecticides to potato psyllid, Bactericera cockerelli (Hemiptera: Psyllidae). Crop Protection 29, 1329–1324.
ACKNOWLEDGEMENTS 2020-10-07
This datasheet was initially prepared in 2013 by J. E. Munyaneza (USDA-ARS, Wapato, US) and extensively revised in 2020 by Riccardo Bugiani & Massimo Bariselli. Their valuable contribution is gratefully acknowledged.
How to cite this datasheet?
Datasheet history 2020-10-07
This datasheet was first published in the EPPO Bulletin in 2013 and revised in 2020, it is now maintained in an electronic format in the EPPO Global Database. The sections on 'Identity', ‘Hosts’, and 'Geographical distribution' are automatically updated from the database. For other sections, the date of last revision is indicated on the right.
EPPO (2013) Bactericera cokerelli. Datasheets on pests recommended for regulation. EPPO Bulletin 43(1), 202-208. https://doi.org/10.1111/epp.12044
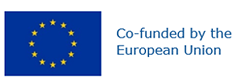