Xanthomonas translucens pv. translucens(XANTTR)
EPPO Datasheet: Xanthomonas translucens pv. translucens
IDENTITY
Authority: (Jones et al.) Vauterin et al.
Taxonomic position: Bacteria: Proteobacteria: Gammaproteobacteria: Lysobacterales: Lysobacteraceae
Other scientific names: Xanthomonas campestris pv. hordei (Hagborg) Dye, Xanthomonas campestris pv. translucens (Jones et al.) Dye, Xanthomonas translucens pv. hordei (Hagborg) Dye
Common names in English: bLS, bacterial leaf streak of barley, bacterial leaf streak of wheat, black chaff of cereals
view more common names online...
Notes on taxonomy and nomenclature
The taxonomy of variants belonging to the Xanthomonas translucens species has been a topic of confusion and debate in the scientific literature, primarily due to the pest’s complex host range infecting many plants within the family Poaceae. This host specificity has contributed to the challenges in accurately classifying and defining this bacterial species. X. translucens exhibits a wide range of strains and variants that infect various cereals and grass species. The overlapping symptoms and pathogenicity observed among different strains infecting these diverse hosts have made it difficult to establish clear taxonomic boundaries. The wide variation in host range has long posed significant question regarding whether these strains represent distinct species or simply different pathovars or subspecies within X. translucens. According to studies utilizing DNA-DNA hybridization, protein electrophoresis, and fatty acid analysis, Xanthomonas strains that were previously known as Xanthomonas campestris pathovars of the 'translucens group' infecting Poaceae were reclassified and grouped within the species Xanthomonas translucens (Vauterin et al., 1992, 1995). These molecular techniques allowed for a more accurate classification and understanding of the relationships between these strains, resulting in the consolidation of the Poaceae-infecting Xanthomonas strains under the species Xanthomonas translucens. X. translucens pv. translucens was classified as strains that caused symptoms when inoculated on barley, but not on other cereals, and includes strains that were previously classified as pv. hordei (Bragard et al., 1995, 1997; Sapkota et al., 2020). Recent advancements in genome sequencing have provided valuable insights into the taxonomy of the X. translucens species. Through comprehensive comparative genomics analysis of whole-genome sequences, researchers have observed that X. translucens can be divided into three distinct clades based on average nucleotide identity (ANI) and digital DNA-DNA hybridization (dDDH) (Goettelmann et al., 2021; Tambong et al., 2023). Pathovar translucens belongs to the clade Xt-I along with the closely related pathovar undulosa. X. translucens pv. translucens is further divided into three main subgroups which are globally distributed (Heiden et al., 2022).
view more categorizations online...
EPPO Code: XANTTR
HOSTS 2024-02-13
While the main hosts of X. translucens pv. translucens are domesticated and wild barley (Hordeum vulgare, Hordeum marinum), occasional asymptomatic isolation from wheat (Triticum aestivum) and rye (Secale cereale) have been recently reported (Curland et al., 2018).
Host list: Hordeum vulgare, Secale cereale, Triticum aestivumGEOGRAPHICAL DISTRIBUTION 2024-02-15
Bacterial Leaf Blight caused by X. translucens pv. translucens was first reported near Madison (Wisconsin, USA) in 1912. The accurate determination of the geographical distribution of X. translucens pv. translucens is challenging due to historical variations in its nomenclature and the misidentification with closely related X. translucens pv. undulosa. The use of different names for this pathogen in the past has led to confusion and inconsistency in the literature, making it difficult to establish a completely accurate and up-to-date understanding of its geographic range. The similarity in characteristics and symptoms between X. translucens pv. translucens and X. translucens pv. undulosa has further complicated the accurate identification and differentiation of these pathogens.
The records given here derive, among other sources, from Bradbury (1986), Duveiller (1989), Duveiller (1994) and are representative of the species X. translucens. Based on the available information, it is believed that X. translucens pv.translucens is present in most barley-growing regions worldwide, with the exception of Western Europe. However, the exact reasons for its absence in Western European barley fields remain unknown. There could be several factors contributing to the absence of the pathogen in Western Europe, but without specific research or studies addressing this issue, it is challenging to determine the exact cause. Some potential factors that could play a role include variations in climate, agricultural practices, or the presence of natural barriers that limit its establishment and spread in the region.
It is noteworthy to mention that recent observations suggest a reemergence of X. translucens pv. translucens within the barley-growing regions of North America (Curland et al., 2018, 2020; Heiden et al., 2022; Beutler et al., 2023; Ritzinger et al., 2023; Tambong et al., 2023; Schachterle et al., n.d.). This resurgence has raised concerns among researchers and farmers, as it highlights the dynamic nature of pathogen populations and their potential to regain prominence in agricultural systems. Efforts are underway to monitor and study the factors contributing to this reemergence, including shifts in environmental conditions, genetic changes in the pathogen, or alterations in host susceptibility. Analysis of diversity parameters such as haplotype frequency, haplotype diversity, and percentage of polymorphic sites revealed significantly higher genetic diversity in Iranian strains compared to those isolated in North American countries (Khojasteh et al., 2019). Moreover, a global-scale phylogeographic analysis supported the hypothesis that Iranian strains of X. translucens have acted as the founding population in other countries, inferring that the centre of origin of the pathogen is from the Middle East and was disseminated in other continents.
Africa: Ethiopia, Kenya, Libya, Morocco, South Africa, Tanzania, Tunisia, Zambia
Asia: China (Henan, Xinjiang), India (Delhi, Rajasthan), Iran, Israel, Japan (Hokkaido), Jordan, Kazakhstan, Malaysia (Sabah), Pakistan, Saudi Arabia, Syria
North America: Canada (Alberta, British Columbia, Manitoba, New Brunswick, Québec, Saskatchewan), Mexico, United States of America (Alabama, Arizona, Arkansas, California, Colorado, Georgia, Idaho, Illinois, Indiana, Iowa, Kansas, Louisiana, Michigan, Minnesota, Mississippi, Montana, Nebraska, North Carolina, North Dakota, Ohio, Oklahoma, Oregon, South Carolina, South Dakota, Texas, Utah, Virginia, Washington, Wisconsin)
South America: Argentina, Bolivia, Brazil (Mato Grosso do Sul, Parana), Paraguay, Peru, Uruguay
Oceania: Australia (New South Wales), New Zealand
BIOLOGY 2024-02-15
Seeds have been reported as the primary source of X. translucens pv. translucens inoculum and large-scale long-distance dissemination of the pathogen (Jones et al., 1916; Jones and Johnson, 1917; Sands et al., 1986; Duveiller et al., 1997). On a local scale, bacteria are transmitted by rain splash, dew and contact between plants (Boosalis, 1951). X. translucens pv. translucens typically enters the host tissue through existing wounds or through natural water pores called hydathodes. These hydathodes are located at the leaf tips and serve as natural openings for the movement of water and dissolved substances in plants. Hydathodes offer a direct route to the water-transporting xylem vessels where bacteria proliferate at high density and cause systemic disease. While the xylem is the main niche of X. translucens pv. translucens, at late stages of infection, bacteria eventually exit the xylem vessels and colonize the intercellular space of the mesophyll followed by the release of bacterial exudate at the leaf surface (Shekhawat and Patel, 1978; Gluck-Thaler et al., 2020). The route from which these exudates are released is currently unclear, but stomata may play an important role in this process. One infected plant can lead to an area of 30 m2 being infected during a growing season (Sands et al., 1986). It has been hypothesized that aphids and other insects that come into contact with these sticky exudates may carry the bacterium and potentially transmit it to nearby plants, aiding in its dissemination (Boosalis, 1952). However, this has not been demonstrated conclusively and remains largely speculative.
The pathogen exhibits remarkable resistance to dry conditions. The organism has been found to survive for up to eight months on straw obtained from blighted plants and can persist for as long as two years within seeds (Jones and Johnson, 1917). It is worth noting that the survival of X. translucens in the field is not solely reliant on the presence of host plants (or host crop residues), as epiphytic populations have been found to persist on non-host species (Timmer, 1987). In the growing season, the optimal temperature range for infection and symptom development is typically between 15 and 22°C (Kim, 1982). The occurrence of disease outbreaks is more common during wet seasons, indicating that warm and humid conditions are critical for the development of the disease (Sapkota et al., 2020). It has been observed that the disease is more prevalent in fields with sprinkler irrigation or during periods of increased rainfall. Inoculation experiments have shown that plants are most susceptible to infection when exposed to moisture, particularly during rainfall or sprinkler irrigation (Sands et al., 1986). It is believed that the presence of moisture on the plant surfaces creates a favourable environment for the bacterium to multiply and establish infections. The wet conditions not only facilitate the survival and multiplication of X. translucens pv. translucens but may also enhance the likelihood of successful pathogen entry and colonization of the host plant. Preliminary analyses of data from previous field trials seems to indicate that the stage of maturity in barley can significantly influence its response to X. translucens pv. translucens.
It has been observed that many barley accessions may exhibit apparent levels of resistance to the pathogen before heading, but become more susceptible to infection once they reach the heading stage (Ritzinger et al., 2023). It has been reported that X. translucens pv. translucens exhibits ice-nucleating activity, which suggests a potential association between the bacterium and frost injury in plants (Kim, 1987; Sands and Fourrest, 1989; Zhao and Orser, 1990). In addition to its potential association with frost injury, the ice-nucleating activity of X. translucens pv. translucens may also play a role in its dissemination (plants are more easily injured and bacteria may be released from these plants and spread e.g. via rain splash).
DETECTION AND IDENTIFICATION 2024-02-15
Symptoms
In the early stages of infection, leaves display distinct symptoms characterized by water-soaked stripe lesions that are prominently visible along the leaf margins when observed under direct light. These lesions appear as areas of abnormal moisture accumulation, giving the affected tissue a soaked and translucent appearance. As the infection progresses, the water-soaked lesions tend to expand in size and merge, forming larger, streak-shaped lesions. Over time, these lesions can undergo necrosis or tissue death. As the disease advances systemically, blight symptoms may emerge on the infected leaves. Blight symptoms often manifest as wilting, yellowing, or browning of the affected tissue, leading to the overall decline of the leaf. Eventually, the entire leaf becomes desiccated. Downstream of the blight, translucent water-soaked lesions can be found within the mesophyll tissue as a result of local infection. The presence of bacterial slime can be observed at the leaf surface, which subsequently dries and forms a thin scale-like layer that can be flaked off. Symptoms in seedlings may be minimal, while severe infections can cause 'black chaff' symptoms in glumes and seeds, characterized by purple-black discoloration of the surface. It typically takes 10-14 days for symptoms to become apparent. The development of water-soaked symptoms followed by tissue death, as well as the subsequent appearance of blight, are typical patterns associated with X. translucens pv. translucens infections. These symptoms serve as important diagnostic indicators for this pathogen.
Morphology
X. translucens pv. translucens bacteria are gram-negative, non-sporing, rod-shaped aerobic, motile, 0.5–0.8 × 1.0–2.5 µm in size, containing a single polar flagellum, and forming typical Xanthomonads yellow colonies on nutrient agar medium (Jones and Johnson, 1917). On Wilbrink's medium, colonies are round, bright, mucoid and yellow (Sands et al., 1986).
Detection and inspection methods
Ideal temperatures for the growth of X. translucens pv. translucens, similar to many other Xanthomonads, are generally around 28°C. Various non-selective media can be utilized to culture the bacterium, such as Nutrient Agar (NA), Wilbrink's medium, Peptone Sucrose Agar (PSA), and King's Broth (KB) (Jones and Johnson, 1917; Sands et al., 1986). However, for isolation, semi-selective media, including modified Wilbrink's medium (seed and leaf tissue) and mTMB (leaf tissue), are preferred (McGuire, 1986; Sands et al., 1986; Duveiller, 1990). Accurately diagnosing the specific pathogens within the X. translucens complex has proven to be a formidable challenge, primarily due to the historical lack of clear taxonomic boundaries surrounding this group and the overlapping host ranges exhibited by these subspecies. As a result, the development of highly specific pathovar-specific diagnostic tools has been lacking. Until recently, detection methods for X. translucens included immunofluorescence microscopy, dot-immunobinding assays, and semi-selective enrichment combined with ELISA (Duveiller, 1992; Frommel and Pazos, 1994). However, these methods are not specific to the pv. translucens (Bragard and Verhoyen, 1993). This lack of specificity is even more critical due to the fact both X. translucens pv. translucens and X. translucens pv. undulosa infect the same host, barley. Inaccurate differentiation between these pathovars can lead to misdiagnosis and the implementation of inappropriate quarantine measure.
PCR tests have recently been developed for X. translucens pv. translucens which have a high sensitivity and can reliably differentiate it from closely related subspecies within the translucens species. A LAMP-based approach has also been developed as a rapid detection and diagnostic method for X. translucens in plant tissues (Langlois et al., 2017). This LAMP assay offers the ability to distinguish between clade Xt-I, Xt clade-II, and Xt clade-III within the X. translucens species (Goettelmann et al., 2021). However, it does not provide specific differentiation between X. translucens pv. translucens and X. translucens pv. undulosa which are both barley pathogens (Xt-1 clade). A probe-based real-time PCR protocol was developed for the detection and quantification of X. translucens pv. undulosa, X. translucens pv. translucens, and X. translucens pv. secalis (Xt-1 clade) (Sarkes et al., 2022). This real-time PCR protocol provides a reliable and sensitive method for detecting the presence of these pathovars; however, it does not differentiate between the three pathovars. In 2022, a rapid multiplex PCR test was developed to detect all subgroups of X. translucens (Xt-I to Xt-III) and differentiate between X. translucens pv. translucens and X. translucens pv. undulosa (Roman-Reyna et al., 2022). Recently, a pathovar-specific TaqMan real-time PCR test was developed with high specificity and analytical sensitivity (Tambong et al., 2023). This test allowed for the specific detection of X. translucens pv. translucens in plant leaf tissue, with the capability to detect as low as 23 CFU mL-1.
Having a reliable and established seed-detection diagnostic method for this seed-borne pathogen is of utmost importance. To ensure the safe trade of agricultural commodities, robust diagnostic tools are needed. Therefore, it is important to highlight that the LAMP assay developed by Langlois et al. (2017) has undergone testing and validation for the detection of the pathogen in barley seeds. In contrast, the study conducted by Sarkes et al. (2022) focused on the detection of X. translucens pv. undulosa specifically in wheat seeds. Even though these approaches do not offer specific differentiation between barley-infecting X. translucens pv. translucens and X. translucens pv. undulosa, their capability to detect both pathogens on seeds makes them valuable and practical seed-detection methods.
PATHWAYS FOR MOVEMENT 2024-02-15
The bacteria are primarily dispersed locally through splashing over short distances. However, the potential for international spread is primarily associated with infected seed lots.
It is crucial to highlight that the presence of a conserved ice-nucleation gene among X. translucens pv. translucens strains indicates its capability for aerial dispersal and long-distance dissemination. Additionally, the isolation of X. translucens from rain events further supports this notion (Failor et al., 2017).
PEST SIGNIFICANCE 2024-02-15
Economic impact
Over the last two decades, X. translucens pv. translucens has re-emerged and raised significant concerns in barley production (Sapkota et al., 2020). Infections caused by this pathogen can have severe adverse effects on both crop yield and quality (Sapkota et al., 2020; Ritzinger et al., 2023). However, there is limited quantitative data regarding the extent of losses caused by this pathogen. This can be attributed to two main factors: the absence of completely resistant barley varieties for comparative analysis and the lack of studies specifically targeting the impacts of X. translucens pv. translucens on barley. Consequently, the understanding of the precise economic and agronomic consequences of X. translucens pv. translucens infections remains relatively limited. Further research and investigations are necessary to fill this knowledge gap.
Control
A combination of management strategies is advisable to control Bacterial Leaf Blight on barley. These include implementing a seed certification program, to ensure the absence of the bacterium in seeds (Forster and Schaad, 1988; Duveiller et al., 1997). Efficient screening and monitoring of seed lots during production, storage, and distribution processes are essential. While there are currently no seed treatments available that provide complete effectiveness against the pathogen, it is possible to implement quarantine measures to prevent the unintended introduction of the pathogen into new regions (Duveiller et al., 1997).
Additionally, the following agricultural practices can be used:
Cultural control
Implementing a crop rotation scheme that includes nonhost plants can help break the disease cycle and reduce the viable inoculum present in the field (Ritzinger, 2022). Furthermore, removing infected crop residue after harvest may help in preventing the survival and spread of the pathogen (Ritzinger et al., 2023). It is also advisable to eliminate potential alternative host weeds located in close proximity to barley fields, as the closely related pathovar X. translucens pv. undulosa was detected on a multitude of weedy grasses typically found around wheat field (Ledman et al., 2021).
Plant resistance
Due to the lack of effective chemical control, host resistance is currently considered to be the most efficient disease management strategy for X. translucens pv. translucens. Consequently, prioritizing germplasm screening for resistance to bacterial leaf blight becomes crucial in management strategies. Previous studies aimed to develop more resilient barley varieties. The resistant cultivar Oderbrucker which was identified as early as 1917 by Jones et al. exhibited resistance in field trials in Montana (Kim, 1982). Three quantitative trait loci (QTLs) associated with resistance to Bacterial Leaf Blight were identified on chromosomes 3 (two QTLs) and 7 (one QTL) of the cultivar Morex, a widely deployed six-row spring malting barley that was released in 1978 by the Minnesota Agricultural Experiment Station (Alizadeh et al., 1994). This cultivar has gained popularity in North American barley fields and serves as the current reference genome for barley. The QTL located near the marker ABG377 on chromosome 3 was found to be the primary contributor to the resistance. However, this resistance is only partial and Morex remains susceptible to the current X. translucens pv. translucens population in North America (Jules Butchacas, personal communication). In a recent study, a panel of 2094 barley accessions was subjected to field trials conducted in Minnesota (Ritzinger et al., 2023). By considering both resistance during leaf development and heading time, the authors were able to narrow down the list of potential Bacterial Leaf Blight-resistant candidates for breeding to 32 accessions. The study also revealed that wild barley parents from countries such as Israel, Lebanon, Iran, Turkmenistan, Azerbaijan, Syria, Türkiye, and Jordan played a significant role in contributing Bacterial Leaf Blight resistance alleles. Wild barley species (e.g. H. spontaneum, H. marinum) have been widely acknowledged for their valuable resistance against various fungal pathogens affecting barley, confirming their potential as a valuable resource for breeding and developing barley varieties with resistance to Bacterial Leaf Blight (Kim, 1982; Dinh et al., 2022). Ritzinger et al., 2023 once again demonstrated the resistance of 'Oderbrucker' to Bacterial Leaf Blight. Ritzinger et al. (2023) underlined the importance of developing genetic markers through genome-wide association studies (GWAS) and classical mapping studies for breeding programs aimed at enhancing Bacterial Leaf Blight resistance in barley. These genetic markers provide essential insights into the underlying genetic mechanisms of Bacterial Leaf Blight resistance and enable targeted breeding efforts.
It is important to acknowledge that the identified germplasm sources resistant to Bacterial Leaf Blight present poor agronomic characteristics. Research should continue to develop varieties that combine resistance to Bacterial Leaf Blight with favourable agronomic characteristics to provide farmers with improved varieties that are resistant to the disease and also possess desirable traits such as high yield potential, good quality, and adaptability to different environmental conditions.
Phytosanitary risk
X. translucens pv. translucens can have negative impacts on the yield of barley, an important crop in the EPPO region. While it is most likely already present within the EPPO region, accurately establishing its exact range poses challenges due to symptom confusion and the historical lack of reliable detection methods. Given its seed-borne nature and its presence in areas with similar climates as in the EPPO region, there is a potential risk for X. translucens pv. translucens to further establish and develop in new parts of the EPPO region if infected seeds are used.
PHYTOSANITARY MEASURES 2024-02-13
To minimize the risk of introducing X. translucens pv. translucens, it is essential to adopt stringent measures for import and exchange of barley seeds. Therefore, robust diagnostic tests and rigorous phytosanitary measures are essential in preventing the unintentional introduction and spread of this pathogen to new areas within the EPPO region. Seeds of host plants should either come from a pest-free area or be certified as pest free if they are produced in countries where the pest occurs. Such measures should also apply to barley seeds imported for breeding purposes in areas where the pest does not occur. To mitigate this risk, it is advisable to produce such seeds in dry and disease-free zones whenever possible, supplemented by regular testing.
REFERENCES 2024-02-15
Alizadeh A, Benetti V, Sarrafi A, Barrault G & Albertini L (1994) Genetic analysis for partial resistance to an Iranian strain of bacterial leaf streak (X. campestris pv. hordei) in barley. Plant Breeding 113, 323–326.
Beutler J, Li T, Roman-Reyna V, Fleitas MC, Bamrah R, Jacobs JM, Kutcher HR, Tambong JT & Brar GS (2023) First report of bacterial leaf streak of barley (Hordeum vulgare) caused by Xanthomonas translucens pv. translucens in British Columbia, Canada. Plant Disease 107(7), 2215. https://doi.org/10.1094/PDIS-09-22-2112-PDN
Boosalis MG (1951) The epidemiology of Xanthomonas translucens (J.J. and R.) Dowson on cereals and grasses. Available at: http://conservancy.umn.edu/handle/11299/224738 [Accessed September 19, 2022].
Boosalis MG (1952) The epidemiology of Xanthomonas translucens on cereals and grasses. Phytopathology 42, 387-395.
Bradbury JF (1986) Guide to Plant Pathogenic Bacteria. CAB International, Wallingford, UK.
Bragard C & Verhoyen M (1993) Monoclonal antibodies specific for Xanthomonas campestris bacteria pathogenic on wheat and other small grains, in comparison with polyclonal antisera. Journal of Phytopathology 139, 193-288.
Bragard C, Verdier V & Maraite H (1995) Genetic diversity among Xanthomonas campestris strains pathogenic for small grains. Applied and environmental microbiology 61(3), 1020-1026.
Bragard C, Singer E, Alizadeh A, Vauterin L, Maraite H & Swings J (1997) Xanthomonas translucens from small grains: Diversity and phytopathological relevance. Phytopathology 87(11), 1111–1117.
Curland RD, Gao L, Bull CT, Vinatzer BA, Dill-Macky R, Van Eck L & Ishimaru CA (2018) Genetic diversity and virulence of wheat and barley strains of Xanthomonas translucens from the Upper Midwestern United States. Phytopathology 108(4), 443-453.
Dinh HX, Singh D, Gomez de la Cruz D, Hensel G, Kumlehn J, Mascher M, Stein N, Perovic D, Ayliffe M, Moscou MJ & Park RF (2022) The barley leaf rust resistance gene Rph3 encodes a predicted membrane protein and is induced upon infection by avirulent pathotypes of Puccinia hordei. Nature Communications 13(1), 2386. https://doi.org/10.1038/s41467-022-29840-1
Duveiller E (1989) Research on 'Xanthomonas translucens' of wheat and triticale at CIMMYT. EPPO Bulletin 19, 97-103.
Duveiller E (1994) Bacterial leaf streak or black chaff of cereals. EPPO Bulletin 24, 135-158.
Duveiller E & Bragard C (1992) Comparison of immunofluorescence and two assays for detection of Xanthomonas campestris pv. undulosa in seeds of small grains. Plant Disease 76, 999-1003.
Duveiller E, Bragard C & Maraite H (1997) Bacterial leaf streak and black chaff caused by Xanthomonas translucens. The bacterial diseases of wheat: concepts and methods of disease management. CIMMYT, Mexico, DF, 25–47.
Failor KC, Schmale DG, Vinatzer BA & Monteil CL (2017) Ice nucleation active bacteria in precipitation are genetically diverse and nucleate ice by employing different mechanisms. The ISME Journal 11(12), 2740–2753. https://doi.org/10.1038/ismej.2017.124
Forster RL & Schaad NW (1988) Control of black chaff of wheat with seed treatment and a foundation seed health program. Plant Disease 72, 935-938.
Frommel MI & Pazos G (1994) Detection of Xanthomonas campestris pv. undulosa-infested wheat seeds by combined liquid medium enrichment and ELISA. Plant Pathology 43, 589-596.
Gluck-Thaler E, Cerutti A, Perez-Quintero AL, Butchacas J, Roman-Reyna V, Madhavan VN, Shantharaj D, Merfa MV, Pesce C, Jauneau A & Vancheva T (2020) Repeated gain and loss of a single gene modulates the evolution of vascular plant pathogen lifestyles. Science advances 6, eabc4516. https://doi.org/10.1126/sciadv.abc4516
Goettelmann F, Roman-Reyna V, Cunnac S, Jacobs JM, Bragard C, Studer B, Koebnik R & Kölliker R (2021) Complete genome assemblies of all Xanthomonas translucens pathotype strains reveal three genetically distinct clades. Frontiers in Microbiology 12, 817815. https://doi.org/10.3389/fmicb.2021.817815
Kim HK (1982) Epidemiological, genetical, and physiological studies of the bacterial leaf streak pathogen Xanthomonas campestris pv. translucens. Doctoral dissertation, Montana State University-Bozeman, College of Agriculture. Available at: https://scholarworks.montana.edu/xmlui/bitstream/handle/1/3685/31762001154135.pdf
Heiden N, Roman-Reyna V, Curland R, Dill-Macky R & Jacobs JM (2022) Comparative genomics of Minnesotan barley-infecting Xanthomonas translucens shows overall genomic similarity but virulence factor diversity (preprint). Genomics. https://doi.org/10.1101/2022.04.05.487151
Jones LR, Johnson AG & Reddy CS (1916) Bacterial blights of barley and certain other cereals. Science 44(1134), 432-433.
Jones LR, Johnson AG & Reddy CS (1917) Bacterial-blight of barley. Journal of Agricultural Research 11, 625-644.
Khojasteh M, Taghavi SM, Khodaygan P, Hamzehzarghani H, Chen G, Bragard C, Koebnik R & Osdaghi E (2019) Molecular typing reveals high genetic diversity of Xanthomonas translucens strains infecting small-grain cereals in Iran. Applied and Environmental Microbiology 85(20), e01518-19. https://doi.org/10.1128/AEM.01518-19
Kim HK, Orser C, Lindow SE & Sands DC (1987) Xanthomonas campestris pv. translucens strains active in ice nucleation. Plant Disease 71, 994-997.
Langlois PA, Snelling J, Hamilton JP, Bragard C, Koebnik R, Verdier V, Triplett LR, Blom J, Tisserat NA & Leach JE (2017) Characterization of the Xanthomonas translucens complex using draft genomes, comparative genomics, phylogenetic analysis, and diagnostic LAMP Assays. Phytopathology 107 (5), 519–27. https://doi.org/10.1094/PHYTO-08-16-0286-R.
Ledman KE, Curland RD, Ishimaru CA & Dill-Macky R (2021) Xanthomonas translucens pv. undulosa identified on common weedy grasses in naturally infected wheat fields in Minnesota. Phytopathology 111, 1114–1121. https://doi.org/10.1094/PHYTO-08-20-0337-R
McGuire RG (1986) Tween media for semiselective isolation of Xanthomonas campestris pv. vesicatoria from soil and plant material. Plant Disease 70(9), 887-891.
Ritzinger M (2022) Identification and genetic characterization of bacterial leaf streak resistance in barley. Thesis, 245 pp. Available at: http://conservancy.umn.edu/handle/11299/227901 [Accessed July 13, 2023].
Ritzinger MG, Smith KP, Case AJ, Wodarek JR, Dill-Macky R, Curland RD & Steffenson BJ (2023) Sources of bacterial leaf streak resistance identified in a diverse collection of barley germplasm. Plant Disease 107(3), 802-808.
Román-Reyna V, Curland RD, Velez-Negron Y, Ledman KE, Gutierrez-Castillo DE, Beutler J, Butchacas J, Brar GS, Roberts R, Dill-Macky R & Jacobs JM (2022) Development of genome-driven, lifestyle-informed markers for identification of the cereal-infecting pathogens Xanthomonas translucens pathovars undulosa and translucens. Phytopathology 113(11), 2110-2118. https://doi.org/10.1094/PHYTO-07-22-0262-SA
Sarkes A, Yang Y, Dijanovic S, Fu H, Zahr K, Harding MW, Feindel D & Feng J (2022) Detection of Xanthomonas translucens pv. undulosa, pv. translucens, and pv. secalis by quantitative PCR. Plant Disease 106(11), 2876-2883. https://doi.org/10.1094/PDIS-03-22-0574-RE
Sands DS, Mizrak G, Hall VN, Kim HK, Bockelman HE & Golden MJ (1986) Seed-transmitted bacterial diseases of cereals: epidemiology and control. Arab Journal of Plant Protection 4, 127-125.
Sands DS & Fourest E (1989) Xanthomonas campestris pv. translucens in North and South America and in the Middle East. EPPO Bulletin 19, 127-130.
Sapkota S, Mergoum M & Liu Z (2020) The translucens group of Xanthomonas translucens: Complicated and important pathogens causing bacterial leaf streak on cereals. Molecular Plant Pathology 21(3), 291–302.
Shekhawat GS & Patel PN (1978) Histology of barley plant and rice leaf infected with Xanthomonas translucens f. sp. hordei. Phytopathologische Zeitschrift 93(2), 105-112.
Tambong JT, Xu R, Fleitas MC, Wang L, Hubbard K & Kutcher R (2023) Phylogenomic insights on the Xanthomonas translucens complex, and development of a TaqMan real-time assay for specific detection of pv. translucens on barley. Phytopathology 113(11), 2091-2102.
Timmer LW, Marois JJ & Achor D (1987) Growth and survival of xanthomonads under conditions non-conducive to disease development. Phytopathology 77, 1341-1345.
Vauterin L, Hoste B, Kersters K & Swings J (1995) Reclassification of Xanthomonas. International Journal of Systematic Bacteriology 45, 472-489.
Vauterin L, Yang P, Hoste B, Pot B, Swings J & Kersters K (1992) Taxonomy of xanthomonads from cereals and grasses based on SDS-PAGE of proteins, fatty acid analysis and DNA hybridization. Journal of General Microbiology 138, 1467-1477.
Zhao JI & Orser CS (1990) Conserved repetition in the ice nucleation gene inaX from Xanthomonas campestris pv. translucens. Molecular and General Genetics 223, 163-166.
ACKNOWLEDGEMENTS 2024-02-13
This datasheet was created by main-author Jules Butchacas and co-authors Nathaniel Heiden and Dr. Jonathan M. Jacobs of The Ohio State University and Dr. Ralf Koebnik of the Plant Health Institute of Montpellier, France. Their valuable contribution is gratefully acknowledged.
How to cite this datasheet?
Datasheet history 2024-02-13
This datasheet was first published in 1997 in the second edition of 'Quarantine Pests for Europe’ and revised in 2023. It is now maintained in an electronic format in the EPPO Global Database. The sections on 'Identity', ‘Hosts’, and 'Geographical distribution' are automatically updated from the database. For other sections, the date of last revision is indicated on the right.
CABI/EPPO (1997) Quarantine Pests for Europe (2nd edition). CABI, Wallingford (GB).
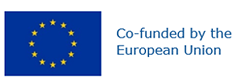